Time to read: 11 min
Ductility, strain, and toughness are fundamental concepts in the field of engineering, particularly in materials science and mechanical engineering.
Ductility is a measure of a material’s ability to deform plastically (permanently) before fracture. In other words, it’s a measure of a material’s ability to stretch or bend without breaking. Ductile materials, such as copper and aluminum, are able to deform a lot before breaking, while brittle materials, such as glass or ceramics, tend to break with very little deformation.
Strain is the deformation of a material per unit length caused by an applied stress. Strain can be thought of as the “amount of stretching” of a material. It is usually expressed as a ratio of the change in length to the original length.
Toughness is a measure of a material’s resistance to fracture when subjected to stress. It’s defined as the energy required to break a material and is typically measured in units of joules per cubic meter (J/m^3). Materials with high toughness are able to absorb a large amount of energy before breaking and are often used in applications where impact resistance is important, such as in construction materials or safety equipment.
Simply put, ductility is a measure of a material’s ability to deform before breaking, strain is the amount of deformation of a material per unit length, and toughness is a measure of a material’s resistance to fracture under stress.
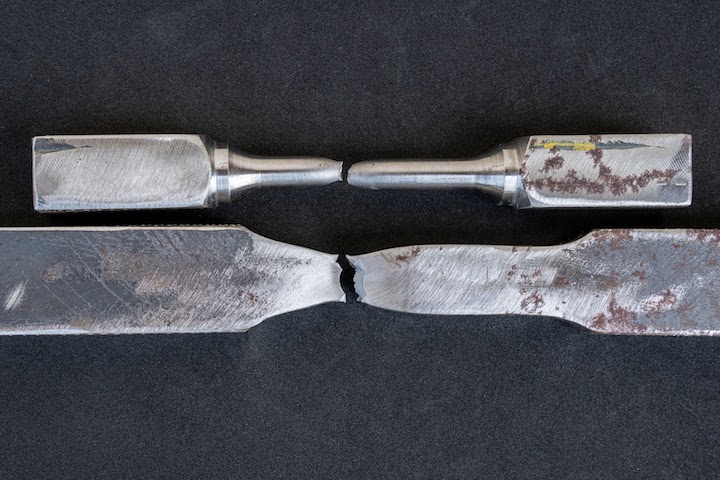
Correlation Between Ductility and Toughness
The relationship between ductility and toughness is complex and depends on several factors, including the type of material, the loading conditions, and the temperature. In general, materials that are more ductile tend to be tougher, as they can absorb more energy before breaking.
However, it’s possible for a material to have high ductility and low toughness or vice versa. For example, some metals, such as aluminum, are both highly ductile and tough, making them well-suited for applications where both properties are important. On the other hand, some materials, such as cast iron, can be very brittle and break with little deformation, even though they have high ductility.
In addition, the relationship between ductility and toughness can also be influenced by the temperature. For example, some materials may become more brittle at low temperatures and less ductile at high temperatures (like ceramics), while others may become more ductile at high temperatures and less brittle at low temperatures (like elastomers).
In general, engineers and materials scientists consider both ductility and toughness when selecting materials for a given application, as both properties play important roles in determining the material’s ability to withstand stress and deformation. The optimal balance between ductility and toughness depends upon the specific requirements of the application.
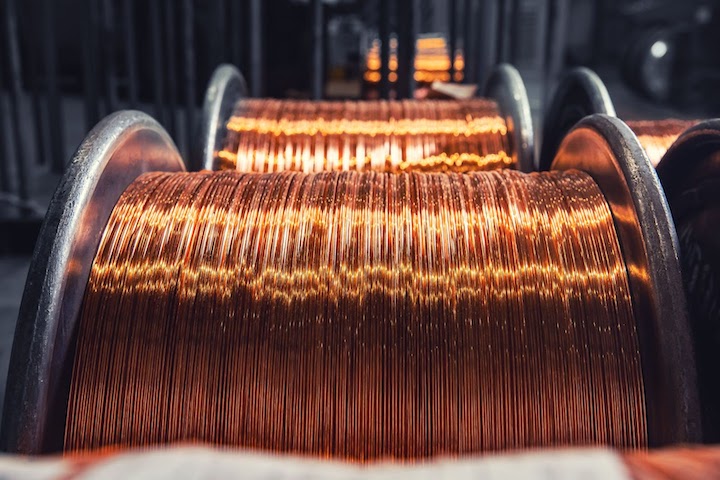
Difference Between Ductility and Toughness
Ductility and toughness are two important properties of materials, but they’re not the same.
Ductility is a measure of a material’s ability to deform plastically (permanently) before fracture. It’s a measure of a material’s ability to stretch or bend without breaking. Ductile materials are able to deform a lot before breaking, while brittle materials tend to break with very little deformation.
Toughness, on the other hand, is a measure of a material’s resistance to fracture when subjected to stress. It is defined as the energy required to break a material and is typically measured in units of joules per cubic meter (J/m^3). Tough materials are able to absorb a large amount of energy before breaking and are often used in applications where impact resistance is important, such as in construction materials or safety equipment.
Ductility is a measure of a material’s ability to deform, while toughness is a measure of a material’s ability to resist fracture under stress. Both properties are important in determining the overall performance of a material in a given application, and the optimal balance between ductility and toughness depends upon the specific requirements of the application.
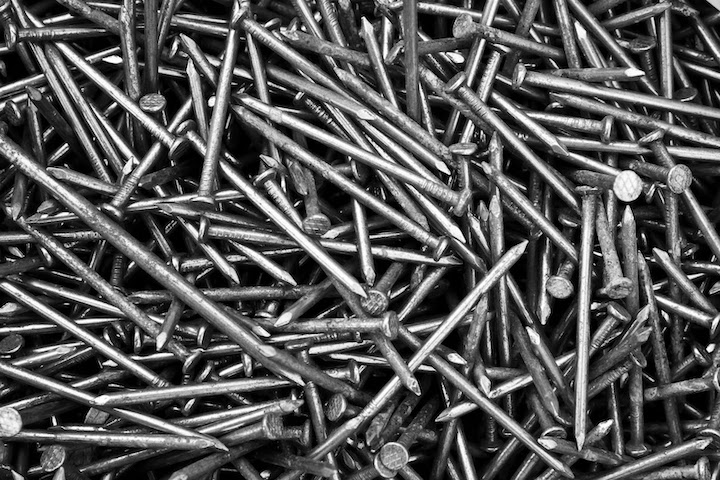
Theory of Mechanical Properties of Materials
The theory of mechanical properties of materials is a branch of materials science that deals with how materials behave when subjected to external loads. This includes the study of how materials deform (elastic deformation and plastic deformation), how they respond to stress and strain, and how they fracture.
The mechanical behavior of materials is determined by the interactions between the atoms and molecules that make up the material. This includes the nature of the bonding between the atoms, the arrangement of the atoms in the crystal lattice, the presence of defects and impurities, and the temperature and environment.
To understand the mechanical properties of materials, engineers and materials scientists use various theories and models, including:
- Linear Elasticity Theory: This theory assumes that the material behaves elastically, meaning that the deformation is proportional to the applied stress and that the material returns to its original shape when the stress is removed.
- Plasticity Theory: This theory considers the plastic deformation of materials, which is the permanent deformation that occurs when a material is subjected to a high level of stress.
- Fracture Mechanics: This theory deals with the prediction of the failure of materials under various loading conditions and is used to understand how and why materials break.
- Fatigue Theory: This theory deals with the behavior of materials under cyclic loading and is used to understand the phenomenon of fatigue and how it can lead to material failure.
- Creep Theory: This theory deals with the slow and continuous deformation of materials under constant stress over time and is used to understand the behavior of materials under high-temperature and long-term loading conditions.
In summary, the theory of mechanical properties of materials is a multidisciplinary field that seeks to understand how materials behave when subjected to external loads, and how these behaviors impact the performance and durability of a material in a given application.
Formula for Toughness
Toughness is a measure of a material’s ability to absorb energy before breaking, and is typically expressed in units of joules per cubic meter (J/m^3). The formula for toughness depends on the type of loading that the material is subjected to.
For tensile loading, the formula for toughness is given by the area under the stress-strain curve. In this case, the toughness is calculated as the integral of the stress-strain curve up to the point of fracture:
T = ∫ (σ dε)
where T is the toughness, σ is the stress, and ε is the strain.
For impact loading, the formula for toughness is given by the energy required to break the material. In this case, the toughness is calculated as the total energy absorbed by the material during the impact test:
T = ∫ (P dt)
where T is the toughness, P is the force applied during the impact, and t is the time.
Toughness is a material property that can be influenced by several factors, including the temperature, the loading rate, the presence of defects and impurities, and the type of loading (tensile or impact). The optimal balance between toughness and other material properties, such as ductility and strength, depends upon the specific requirements of the application.
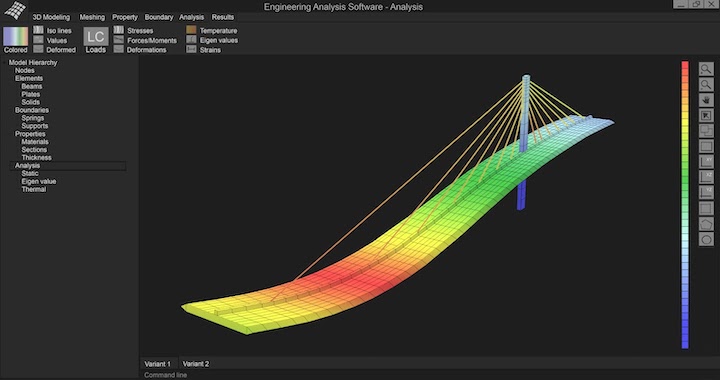
High Toughness Vs High Ductility?
High toughness and high ductility are both desirable material properties, but they’re not directly correlated. A material can have high toughness without being highly ductile, and vice versa.
High toughness means that a material is able to absorb a lot of energy before breaking, making it suitable for applications where impact resistance is important, such as in construction materials or safety equipment.
High ductility means that a material is able to deform plastically (permanently) before fracture, making it suitable for applications where a material needs to bend or stretch without breaking, such as in wire or sheet metal.
In some cases, a material may have both high toughness and high ductility. For example, some steels and alloys have a combination of high strength, high ductility, and high toughness, making them suitable for a wide range of applications.
In summary, high toughness and high ductility are not directly correlated, but both properties are important in determining the overall performance of a material in a given application. The optimal balance between toughness and ductility depends on the specific requirements of the application.
Difference Between Strain and Ductility
Strain and ductility are related concepts in materials science and engineering, but they refer to different aspects of a material’s behavior under stress. Strain is a measure of the deformation of a material caused by an applied stress. It is defined as the change in length of a material divided by its original length:
ε = ΔL / L0
where ε is the strain, ΔL is the change in length, and L0 is the original length.
Ductility, on the other hand, is a measure of a material’s ability to deform plastically (permanently) before breaking. It’s a measure of a material’s malleability, or its ability to be stretched or bent without breaking. Ductility is often expressed as a percentage elongation, which is the increase in length of a material divided by its original length after fracture:
% elongation = (Lf – L0) / L0 * 100%
where Lf is the length of the material after fracture.
In summary, strain is a measure of the deformation of a material caused by an applied stress, while ductility is a measure of a material’s ability to deform plastically before breaking. The two concepts are related in that a material’s ductility is related to the amount of strain that it can undergo before breaking.
Factors That Affect Toughness
There are several factors that can affect the toughness of a material, including:
- Composition: The chemical composition of a material can have a significant impact on its toughness. For example, the addition of certain elements to a material, such as carbon in steel, can increase its strength, but decrease its toughness.
- Microstructure: The size, shape, and distribution of the microstructural features within a material, such as grain size, second phases, and inclusions, can affect its toughness. A fine-grained microstructure, for example, can lead to a more uniform distribution of stress and a higher toughness compared to a coarse-grained microstructure.
- Temperature: The temperature at which a material is loaded can affect its toughness. At high temperatures, a material’s toughness can decrease due to the reduction in its strength and the increase in its ductility.
- Loading rate: The rate at which a material is loaded can affect its toughness. At high loading rates, a material’s toughness can decrease due to the reduction in its ductility.
- Environment: The environment in which a material is used can affect its toughness. For example, exposure to corrosive environments, high temperatures, and radiation can all reduce the toughness of a material.
- Processing: The processing history of a material, such as casting, forging, or heat treatment, can affect its toughness. For example, certain heat treatments can improve the toughness of a material by refining its microstructure.
In conclusion, the toughness of a material is influenced by a combination of its chemical composition, microstructure, temperature, loading rate, environment, and processing history. The optimal balance between toughness and other material properties, such as strength and ductility, depends on the specific requirements of the application.
Does Toughness Increase with Hardness?
Hardness and toughness are two distinct material properties that can be influenced by different factors, and an increase in hardness does not necessarily correspond to an increase in toughness.
Hardness is a measure of a material’s resistance to indentation, and it is often used as an indicator of a material’s strength. Hardness can be increased by increasing the strength of the material, such as by adding elements to a steel alloy.
Toughness, on the other hand, is a measure of a material’s ability to absorb energy before breaking. It is a combination of strength and ductility, and it is often used as an indicator of a material’s impact resistance.
In some cases, increasing the hardness of a material can actually reduce its toughness. For example, adding elements to a steel alloy to increase its strength can also increase its hardness, but it can also lead to a decrease in ductility and toughness.
In general, the optimal balance between hardness and toughness will depend on the specific requirements of the application. For applications where impact resistance is important, a material with high toughness may be preferred, even if it has a lower hardness. For applications where high hardness is required, a material with high hardness may be preferred, even if it has a lower toughness.
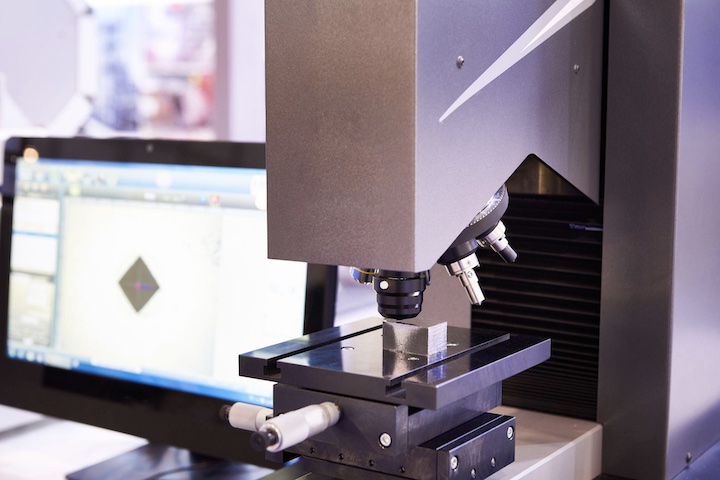
How Is Strain Related to Ductility?
Strain and ductility are related concepts in materials science and engineering that describe a material’s behavior under stress. Strain is a measure of the deformation of a material caused by an applied stress. It’s defined as the change in length of a material divided by its original length:
ε = ΔL / L0
where ε is the strain, ΔL is the change in length, and L0 is the original length.
Ductility, on the other hand, is a measure of a material’s ability to deform plastically (permanently) before breaking. It’s a measure of a material’s malleability, or its ability to be stretched or bent without breaking. Ductility is often expressed as a percentage elongation, which is the increase in length of a material divided by its original length after fracture:
% elongation = (Lf – L0) / L0 * 100%
where Lf is the length of the material after fracture.
Strain and ductility are related in that they both describe the deformation of a material under stress. A material with high ductility can deform more before breaking, which means that it will be able to withstand a larger strain before breaking. Conversely, a material with low ductility will deform less before breaking, which means that it will be able to undergo a smaller strain before breaking.
In summary, strain is a measure of the deformation of a material caused by an applied stress, while ductility is a measure of a material’s ability to deform plastically before breaking. The two concepts are related in that a material’s ductility is related to the amount of strain that it can undergo before breaking.
How to Find Resilience from the Stress Strain Curve
Resilience is a measure of a material’s ability to absorb energy before it yields, or becomes permanently deformed. In the context of a stress-strain curve, resilience can be calculated as the area under the stress-strain curve up to the yield point.
The yield point on the stress-strain curve is the point at which the material begins to yield, or experience permanent deformation. The resilience of the material is equal to the amount of energy required to deform the material to the yield point.
To find the resilience of a material from its stress-strain curve, you can use the formula:
R = (1/2) * σy * εy
where R is the resilience, σy is the yield stress, and εy is the yield strain.
The yield stress and yield strain can be obtained from the stress-strain curve, and the resilience can be calculated as the product of the yield stress and yield strain divided by two.
In general, a material with high resilience will be able to absorb more energy before yielding, which means that it can withstand a greater amount of stress before becoming permanently deformed. Conversely, a material with low resilience will be able to absorb less energy before yielding, which means that it can withstand a smaller amount of stress before becoming permanently deformed.
Complex Parts at Ridiculous Speeds – Start Your Next Project With Fictiv
Whether it’s CNC machining, injection molding, 3D printing or urethane casting, Fictiv is your operating system for custom and complex mechanical parts. Our engineering materials science experts can help you pick the right material for your application, and guide you through the manufacturing process so that you get great results.
Create an account and upload your design today to see what our instant online CNC quote process, DFM feedback, and intelligent platform can do for you — we deliver complex parts at ridiculous speeds!