Time to read: 9 min
Friction is one of the five contact forces along with tension, spring force, normal force, and air resistance. Friction is what gives us traction on the road, good brakes, and allows us to walk without looking like a baby giraffe. But sometimes we just want to let things slide, and that’s when friction frustrates — engines seize up, stuck bolts, and old grandfather clocks frozen, never to chime again. Now, as engineers, we absolutely need friction (and sometimes we need less of it), but we also need to design for it to be consistent.
When dealing with gears, we want parts to slide over each other easily. But in the same assembly we may need two parts to move smoothly using controlled friction. So, how do you control the friction in your assembly of moving parts? First, let’s start with a physics lesson and review the basics of friction.
How Does Friction Work?
In order to control friction, we must understand it. Friction results from two bodies interacting at their contact surfaces — it’s the force that tries to resist movement between those two bodies. There are two types of friction: static and kinetic.
The maximum friction force for either case is defined by:
In this equation, μ is the friction coefficient that is a constant proportional to the “stickiness” of the two bodies, and is dependent on materials and surface finishes. For example, this coefficient is very high between rubber and rough concrete, but very low between steel and teflon.
FN is the normal force, or the amount of force pushing the two bodies closer together — often due to gravity or the weight of the object on the other. It’s a simple concept, except that the friction constant is different when the bodies are moving versus when they’re stationary against each other.
If you’ve ever pushed really hard against a box, only to have it suddenly lurch forward leaving you flat on the ground, you’ve performed an intuitive (albeit painful) experiment. That experience proves that as long as two parts are stuck against each other, their coefficient of friction, or static coefficient of friction (μstatic ,often abbreviated μs ) is higher than the kinetic coefficient of friction, μk, which is a constant used to measure the resistance between two objects when they are moving against each other (as long as you stay above the atomic level).
So, technically, the maximum force due to friction can be calculated as:
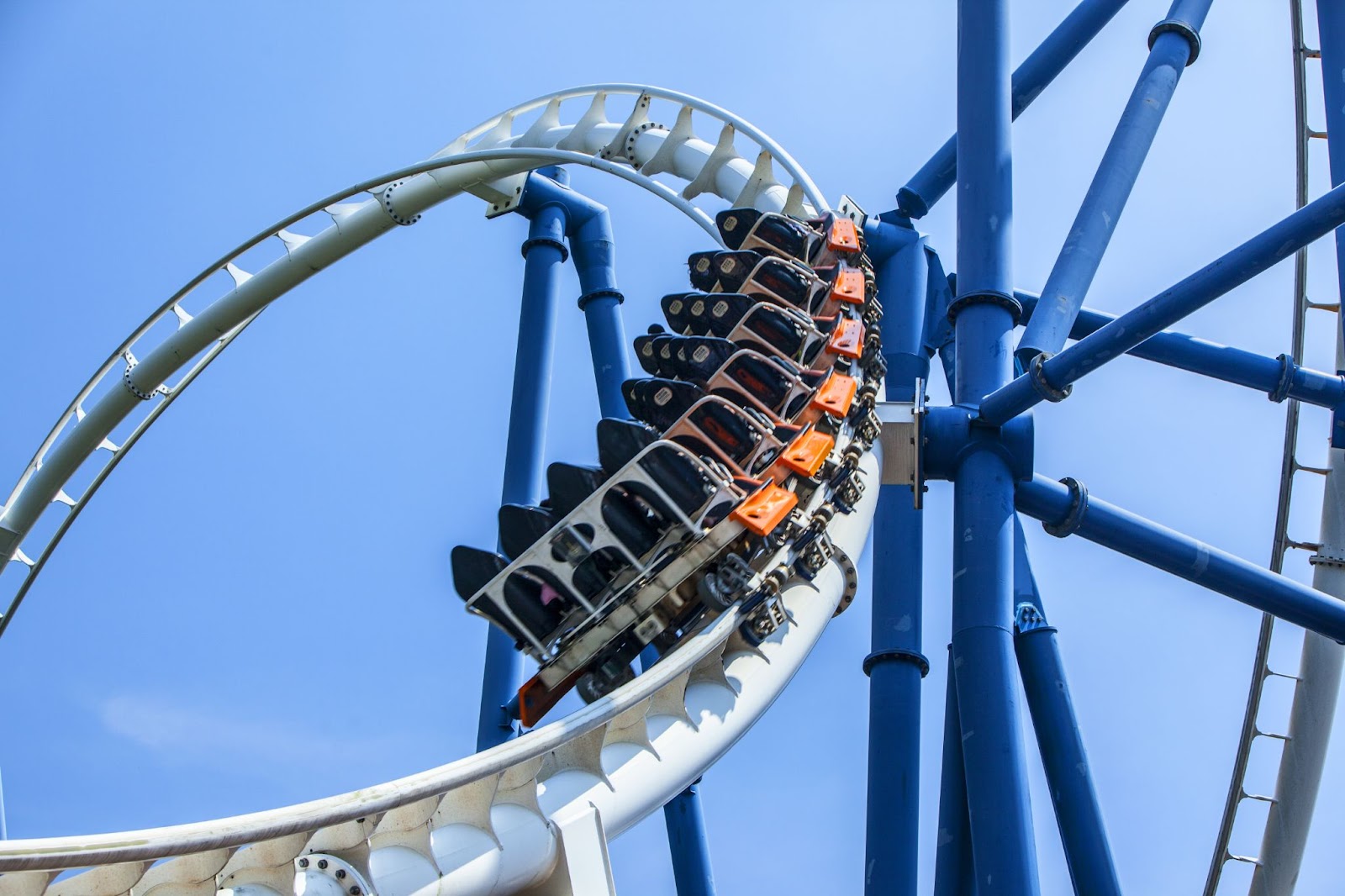
Static friction is essential for traction and is what keeps us from sliding around when we’re walking. As long as two bodies aren’t moving against each other — even when a force is applied — then the relationship between the force required for movement and the static force looks like this:
Once the force trying to slide the parts against each other becomes great enough to overcome the static coefficient of friction, the parts begin to slide, but not smoothly. Because the kinetic friction is less than static, the moving body will suddenly accelerate.
The kinetic friction equation looks similar to static:
However, kinetic friction has a lower coefficient of friction because the coefficient of kinetic friction is always less than the coefficient of static friction:
For most scenarios, you don’t want to deal with the transition between these two, because of the sudden lurch that occurs when kinetic friction becomes dominant. Unfortunately, a common design issue is “stick-slip”, in which a mechanism alternates between the two friction states.
Stick-Slip Scenarios
Stick-slip is fairly easy to understand, but the issue can have dire consequences. For an easy example, consider a car trying to stop in winter. Let’s say you’re driving on dry pavement, and see an intersection coming up. You apply the brakes and the brake pads grip the discs with kinetic friction, allowing the discs to slide by in a controlled way. Meanwhile, the tires grip the pavement with static friction, which provides you control and traction.
…Until you hit a patch of ice in your old Jeep without anti-lock brakes.
Your brakes lock up and shift from kinetic to static friction, while the tires do the opposite — losing traction and moving to low-resistance kinetic friction. You release the brakes, hoping to restore the static friction of the tires with the road. As long as the ice is relatively uniform, you’ll probably be fine.
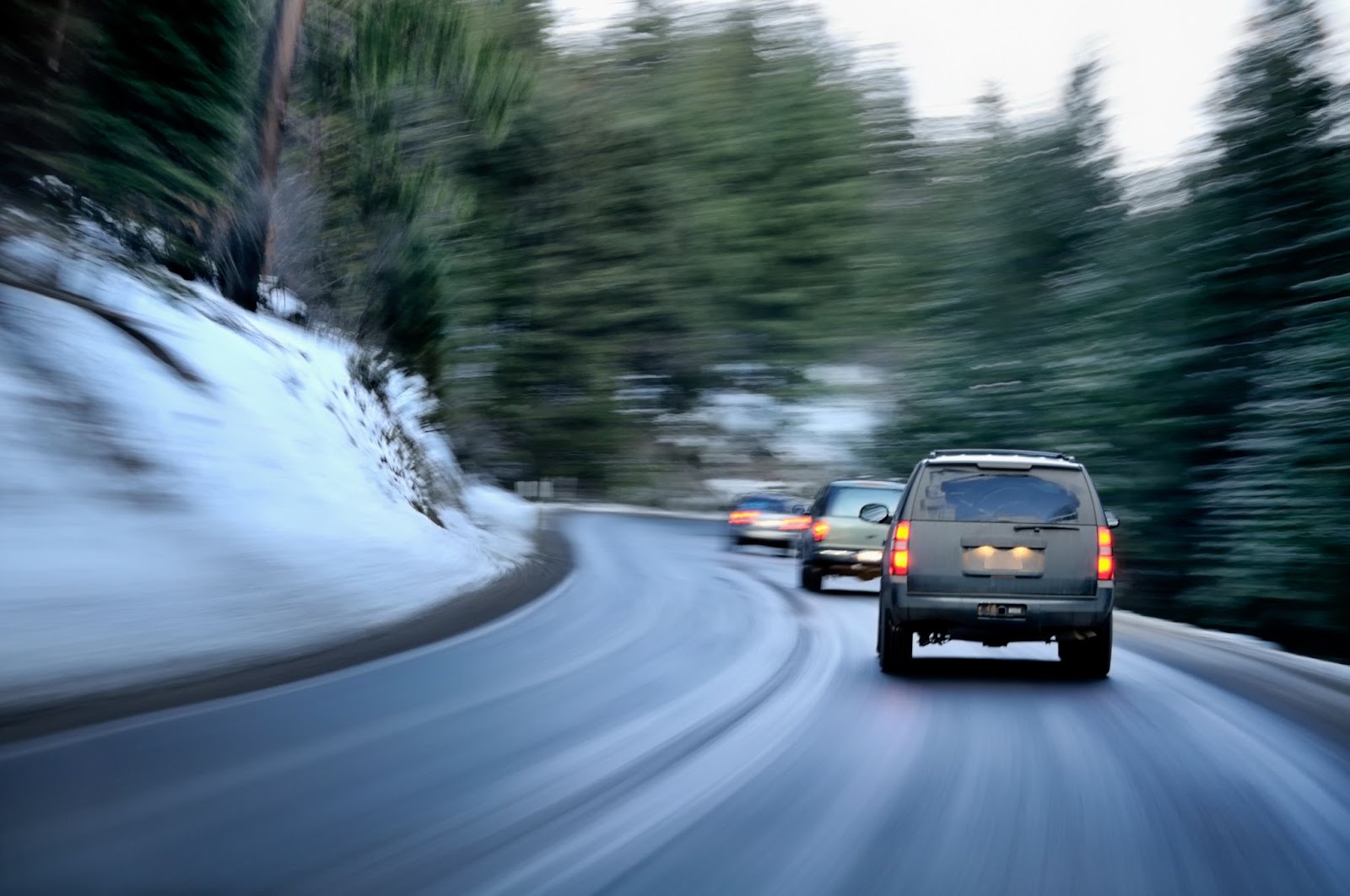
However, if you hit a patch of dry pavement while sliding sideways, you’re in trouble — the tires sudden grip on pavement is likely to flip your car. Granted, that’s an extreme example of how troublesome stick-slip can be, but it shows the importance of figuring out how to avoid stick-slip scenarios!
Avoiding Stick-Slip Scenarios
The easiest way to prevent the dreaded stick-slip is to design your geometry to avoid situations with quickly fluctuating forces, which are often caused by over-constraint or misalignment.
Consider this common design scenario: a machine part is guided along two parallel rails. At first glance, this seems like a great design. The two rails ensure alignment, and with some decent bushings, the moving part should glide along effortlessly. Right?
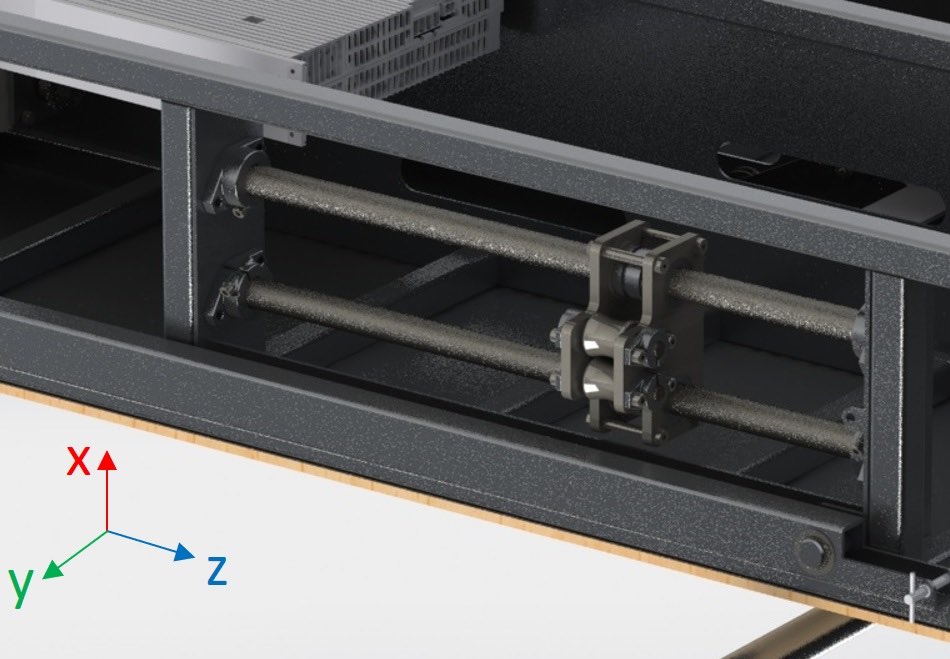
Not quite. Let’s look at the degrees of freedom (DOFs) at play. The first rail allows movement in the z-direction and restricts movement along the x and y axes. It also allows rotation about the z axis, but restricts rotation about the x and y axes. The first rail eliminates four DOFs, and allows movement in two — and our goal is to have movement in only one DOF (along the z axis). So far, so good.
Then we introduce a second rail that also tries to eliminate freedom in the same four DOFs. The result is an overconstrained assembly — the two rails will never be perfectly aligned. A better solution is to use one round rail to constrain four DOFs and a second rail with wheels on either side to eliminate the remaining DOF rotation along the z-axis.
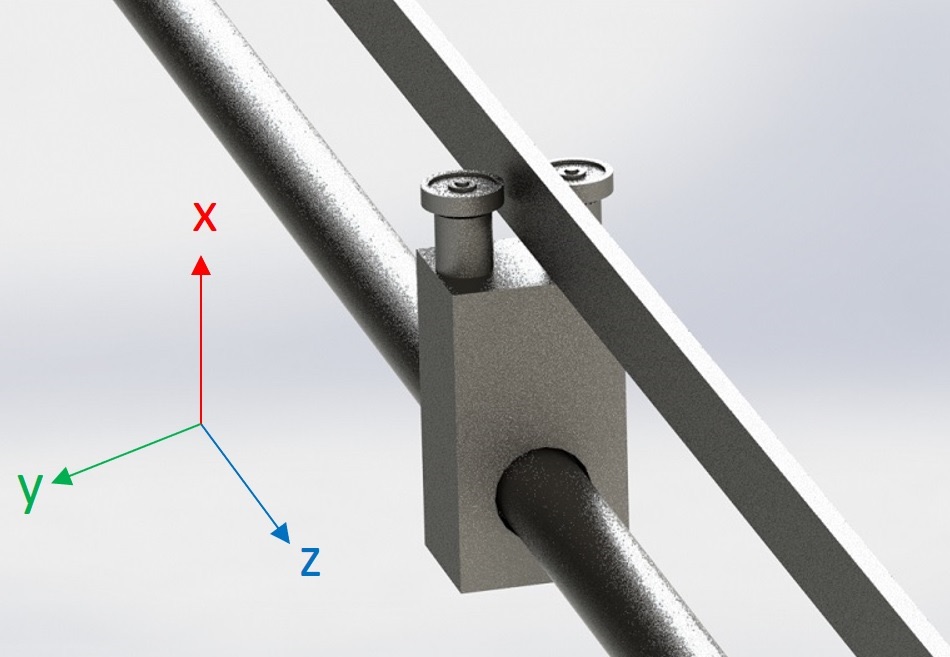
Once you’ve designed your geometry to maximize alignment and eliminate over-constraint, you’re ready to consider other ways of reducing the friction between parts.
Self-Lubricating Materials for Kinetic Friction
Of course, the best way to reduce friction between moving parts in mechanical designs is to incorporate some type of roller bearing. However, that’s an expensive (and therefore impractical) solution for inexpensive consumer designs — and they don’t work for very small spaces, either. Luckily, parts made from self-lubricating materials are nearly as good for a fraction of the price.
A quick glance at coefficients of friction shows that not all materials are created equal when it comes to friction matings. Rubber on concrete is incredibly high — a coefficient of 1.0 – while teflon against teflon is only 0.04. Even for materials with middle-of-the-pack coefficients, the numbers typically halve when lubricant is introduced.
So, how can you take advantage of these disparities?
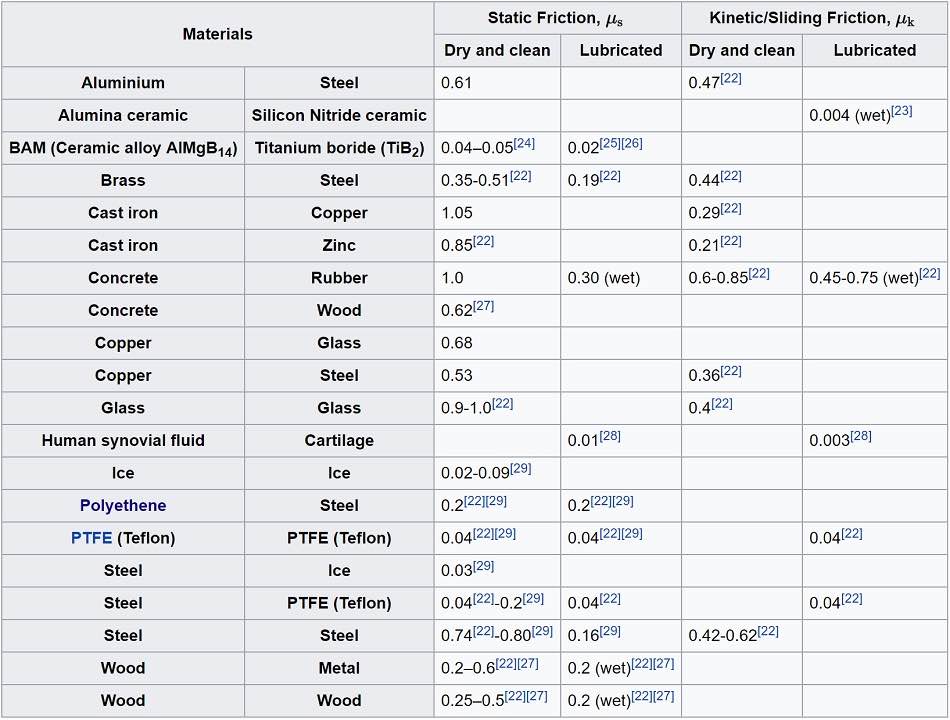
The most straightforward solution is to look for mating materials with a naturally low coefficient of friction, and incorporate them into designs whenever possible. Obviously, Teflon and Delrin are always low-friction material options, but other plastic materials work well, too. Nylon is low-friction against many other plastics, as well as metals, and costs less. Plus, with low-friction plastics or plastic-to-metal matings, you can avoid lubrication in many designs.
However, some situations require greater strength, and metal bushings are are a good solution. Bronze is a common choice for mating against steel, and it’s possible to have bronze with lubricant (such as silicone) impregnated right into the material — providing strength and exceptionally low friction without the need for constant lubrication. Impregnated metals come in many varieties and are an excellent choice for situations requiring strength and low friction.
But even with very low-friction materials, you should carefully consider the impact of surface finish on the contact surfaces. On the macroscopic level, a majority of the friction force is due to surface characteristics, so smoother surfaces have less friction — but if the surfaces are too smooth, they’re likely to squeak and wear out quickly. In general, metal surfaces of 32 – 64 rms will be very low-friction (and easily milled), while surfaces around 20 rms (easy for turned parts) will have a longer life.
When even greater strength or smoother operation than bushings is required, it’s a good idea to consider caged bearings, such as ball or pin bearings. However, with some creative design, the need for sliding parts may be eliminated altogether…
Using Flexure in Place of Sliding Parts
Before plastics were common, industrial designs had an amazing number of pins, joints, rails, and slides. Growing up, my dad’s blacksmithing shop had a hundred-year-old industrial automatic power hammer, and the only way to keep it running was to dump half a can of grease into the dozen Zerks lubricating all the moving parts. However, since the advent of polymers in the last half of the 20th century, we’ve enjoyed significantly more flexibility in our designs.For instance, take a look at an old-style candy container, like an Altoids tin. Even on a cheap container like this, the tin is carefully folded around to make a hinge, creating a small, high-friction joint. Now, take a look at a Bubble Yum container (man, haven’t thought about these for a while) or a floss box, and notice the complete lack of sliding parts. A living hinge across the back has replaced friction with flexure.
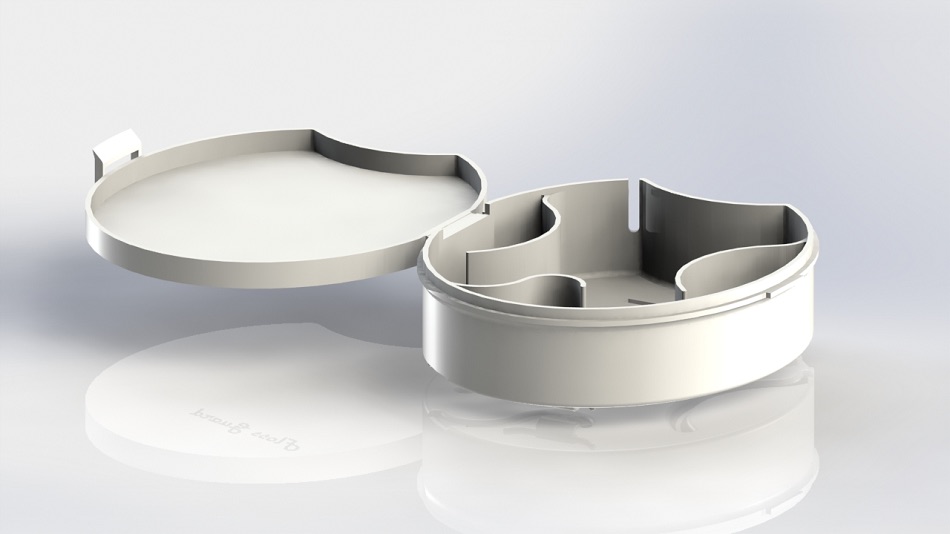
Similarly, latches on old cabinets or enclosures used to be complex and friction prone, where an axle support, pin, latch and spring were all part of the design. Current designs replace that complexity with simple cantilever latches, which eliminates friction points and a great deal of cost.
Of course, with any design, you can’t really trust it until you’ve built a prototype — and these days there are some great, cost-effective ways to test your designs.
Material Selection for Low Friction Mechanical Assemblies
Most bushings — whether plastic or metal — and bearings are standard parts available off the shelf. So, they can be inserted into CNC machined or 3D-printed prototypes, letting you test real geometry and materials in full. Few reasons exist for customizing bearings and bushings, so take advantage of mass production to lower project costs.
For designs that require flexible plastics, prototyping is more complex. Many 3D printed materials are brittle and cannot withstand repeated flexure, so choose your material and printing method carefully.
In basic, low-cycle tests, PLA and TPU printed with FDM can be used to create good-quality living hinges. SLS in Nylon is another good choice for repeated flexure, while most PolyJet materials are too brittle for many cycles — however, new PolyJet materials are produced all the time, and some, like TangoPlus, work well in flexible applications. (For the latest 3D Printing materials, check the Fictiv Capabilities Guide and our Materials Guide.)
Application to Your Design
Whatever material and geometry you choose for your design, you should keep in mind the basics of dynamic designs — looking at degrees of freedom and examining ways to lower friction. We’ve come along way from beef tallow on wagon axles, but the basics haven’t changed since that era. Some of the most beautiful parts of mechanical design are the constants, the ways in which we can apply old principles to new designs.
Sourcing Simplified – Start Your Next Project With Fictiv
Want to learn more about choosing materials for your next project? Check out our guide to material data sheets to learn what’s best for your application.
And if you’re tasked with sourcing and supplying custom parts for any assembly (lubricated or not), Fictiv is your operating system for custom manufacturing that makes part procurement faster, easier, and more efficient.
In other words, Fictiv lets engineers, like you, engineer. Create an account and upload your part to see what our instant quote process, design for manufacturability feedback, and intelligent platform can do for you. Our CNC machining service can create quality precision parts in as little as 2 days!